Developmental Biology
Research interest
Our group is interested in the developmental biology of higher vertebrates. We would like to understand how the vertebrate body is generated during embryogenesis. Starting off as a single cell, the embryo acquires progressively complex traits, including multiple cell types, multiple tissue types, and their species-specific arrangement in functional organs which cooperate to form the developing organism. We are investigating the cellular and molecular mechanisms regulating this fascinating process, including cell fate choice, cell migration, pattern formation and morphogenesis. Beyond embryogenesis we are searching for clues from developmental processes to understand the evolution of vertebrate anatomy.
Model systems and techniques
We mainly use the chicken embryo as a model system, as it allows us to combine classical techniques like microsurgery and immunohistochemistry with modern molecular approaches like local transfection of embryonic cells by in ovo electroporation and retroviral gene delivery, and visualization of the expression of developmentally relevant genes by in situ hybridization.
1. 2,5 days old chicken embryo in ovo (unpublished original)
2. 4 days old chicken embryo stained for muscle specific gene expression (MyoD) in the myotomes by in situ hybridization (unpublished original)
3. Muscle precursor cells emigrating from the somites into limb bud labelled by GFP electroporation, visualized by confocal microscopy (Scaal et al. 2004)
1. Three myotomes of a 5 days old chicken embryo labelled by GFP electroporation, visualized by confocal microscopy (unpublished original)
2. Quail-chicken-chimerization: After transplantation of a ventral somite half of a quail donor into a chicken host, donor cells (green) are found in sclerotomal anlagen of the vertebral column and in the wall of the dorsal aorta (red) (Wiegreffe et al. 2007)
Our research is focussed on the development of the somites. Somites are transient structures found in all chordate embryos. They form a series of mesodermal segments along the body axis, and give rise to a variety of mesodermal organs including the axial skeleton, skeletal muscle, connective tissue of the trunk, and blood vessels. We are studying the molecular regulatory networks and cellular processes underlying the cell fate decisions within the somites, and the subsequent developmental steps leading to the differentiation of somite-derived cells into tissues and organs.
Presently, we are investigating the following questions:
What is the role of signalling filopodia in somite cell fate choice and differentiation?
Somites are initially formed as epithelial spheres, which undergo epithelial-mesenchymal transition (EMT) in the ventral subcompartment to form the sclerotome, which gives rise to the vertebral column. The dorsal somite compartment remains an epithelial cell sheet, the dermomyotome, with its basal pole on the dorsal side facing the ectoderm, and its apical pole on the ventral side facing the sclerotome. Due to individual cell fate decisions which are still not well understood, a fraction of dermomyotomal cells undergo EMT and migrate ventrally between dermomyotome and sclerotome, where they form the primitive musculature of the embryo, the myotome. Another fraction of dermomyotomal cells also undergo EMT, but migrate dorsally to from the dermal and subcutaneous connective tissue of the back.
On a molecular level, it is known that EMT in the dermomyotome is regulated by Wnt signaling from the neural tube and the ectoderm (Geetha-Loganathan et al. 2006, Krück and Scaal 2012).
We are interested in the role of long filopodia-like protrusions, or signaling filopodia, in long range signaling events during dermomyotomal differentiation. Interestingly, we could show that these signaling filopodia show retrograde membrane flow and retrograde transport of the Wnt-receptor Frizzled7 (Sagar et al. 2015, Pröls et al. 2016). We are presently characterizing the cytoskeletal architecture, molecular regulation, and function of these signaling filopodia during somite development.
Epithelial somite showing filopodia-like protrusions extending towards the ectoderm. Green: Actin-GFP, Blue: DAPI-stained nuclei. (unpublished original).
Close-up of somite cells carrying filopodia-like protrusions after transfection with Tubulin-GFP (green). Blue: DAPI stained nuclei. (Sagar et al. 2015)
How do somite cells form the ventrolateral body wall?
In vertebrate embryos, the ventrolateral body wall, i.e. the thorax and the abdominal wall, are formed by immigration of somite cells into the mesenchyme of the lateral plate mesoderm. Specifically, intercostal and abdominal muscle cells are formed from the hypaxial myotomes which are thought to extend laterally into the resident mesenchyme, and the anlagen of the ribs are extensions of the lateral sclerotome domain which converge at the sternal anlage in the ventral midline. The molecular and morphogenetic events guiding these processes are largely unknown. We investigate the lateral extension movements of rib, intercostal muscle and abdominal muscle anlagen to provide a basis for studies on the molecular regulation of these morphogenetic steps in embryogenesis.
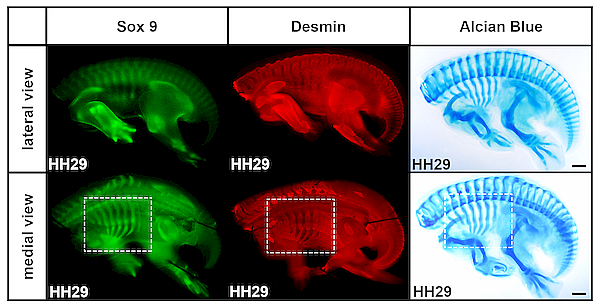
Thorax development in the chicken embryo illustrated by Sox9 staining labelling cartilage anlagen, Desmin staining labelling muscle anlagen, and Alcian Blue staining labelling cartilage. From Khabyuk et al. 2022.
ER-resident chaperones control development and tissue integrity
Proper embryonic development as well as the maintenance of cellular structures entirely depend on a closely controlled communication between cells and cellular layers. Epithelial borders have to be respected while growth and tissue repair require the transient mesenchymalization of epithelial tissue (EMT, epithelial-mesenchymal- transition) but also the epithelialization of mesenchymal tissue (MET, mesenchymal-epithelial transition). EMT and MET are controlled by secreted ligands that bind to receptors at the plasma membrane of the responding cell. Proteins destined to be inserted in the plasma membrane (such as receptors) or to be secreted into the extracellular space (such as ligands, growth factors, extracellular matrix proteins) have to transit the endoplasmic reticulum (ER), where they are posttranslationally modified and subjected to a quality control system. Proteins that do not pass the quality control system are retrotranslocated to the cytosol to be degraded by the proteasome machinery. Accordingly, the chaperone machinery in the endoplasmic reticulum (ER) is crucial for controlling protein synthesis, protein maturation, protein transport and protein degradation. We showed that a member of the ER-resident chaperone machinery, the Mdg1/ERdj4 co-chaperone, is highly expressed during early embryonic development but also during wound healing in chronic wounds. The regional distribution of the Mdg1/ERdj4 protein during embryogenesis points to its role in maintaining the epithelial borders thereby controlling EMT (Daverkausen-Fischer et al. 2020).
Fig. 1: During embryonic development, the regional protein levels of the cochaperone Mdg1/ERdj4 are focused to the epithelial borders and suspected to be crucial for maintenance of the epithelial state of the cells (Daverkausen-Fisher et al. 2020).
Also in chronic wounds, the endothelial linings express high amounts of Mdg1/ERdj4 (Fig. 2,Tsaryk et al. 2015) and we could show that the chaperone pattern is largely dysregulated in malignant vascular tumors while it resembles the embryonic pattern in hemangiomas (Anspach et al 2020).
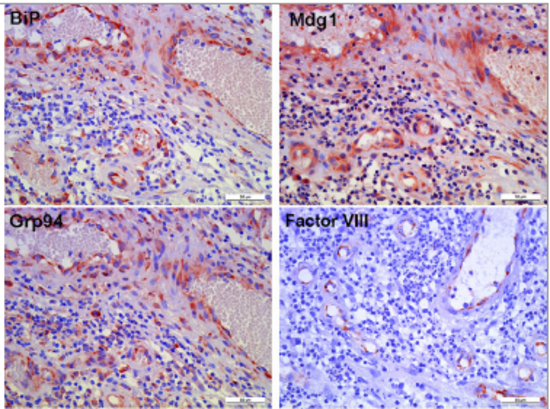
Fig. 2: Immunohistochemical staining of BiP, Mdg1 and Grp94 in dermal chronic wound tissue. (a) Endothelial cells, characterized by factor VIII staining, are positive for BiP, Mdg1/ERdJ4 and Grp94 in inflamed tissue (Tsaryk et al. 2015).
Members of the ER-resident chaperone machinery are not exclusively located in the ER. Recent data show that “ER-resident” chaperones are also located in the cytosol, the nucleus and they are even found to be secreted into the extracellular space. Due to their manifold locations and functions it is not suprising that non-functional chaperones are involved in a hugh variety of metabolic diseases. Several of these aspects have recently been summarized in a series of review articles.
Clinical Anatomy
In collaboration with the Department of Traumatic and Orthopedic Surgery in Cologne, the Department of Pediatrics and other clinical departments of the University of Cologne we are engaged in biomechanical studies and investigations of novel surgical techniques with a special focus on surgery in the locomotory system, as well as intensive care preocedures in newborns. Thanks to the body donation program of the Cologne Center of Anatomy, we are able to perform these studies using authentic human material, which enables us to acquire results of high clinical relevance and applicability. We are grateful to to our body donators who thus allow us to contribute to the scientific progress in m sciences to the benefit of patients.
Testing setup to measure peri-implant failures after plate application to a human humerus. Collaboration with Department of Traumatic and Orthopedic Surgery (Hackl et al. 2015).